Scientists Demote a Potential Wonder Quantum Material
An international research team, including UT Austin's Edoardo Baldini, has disproven that Ta2NiSe5 is an excitonic insulator, resolving a long-standing debate on its phase transition and showing that structural, not electronic, changes inhibit superfluid energy transport.
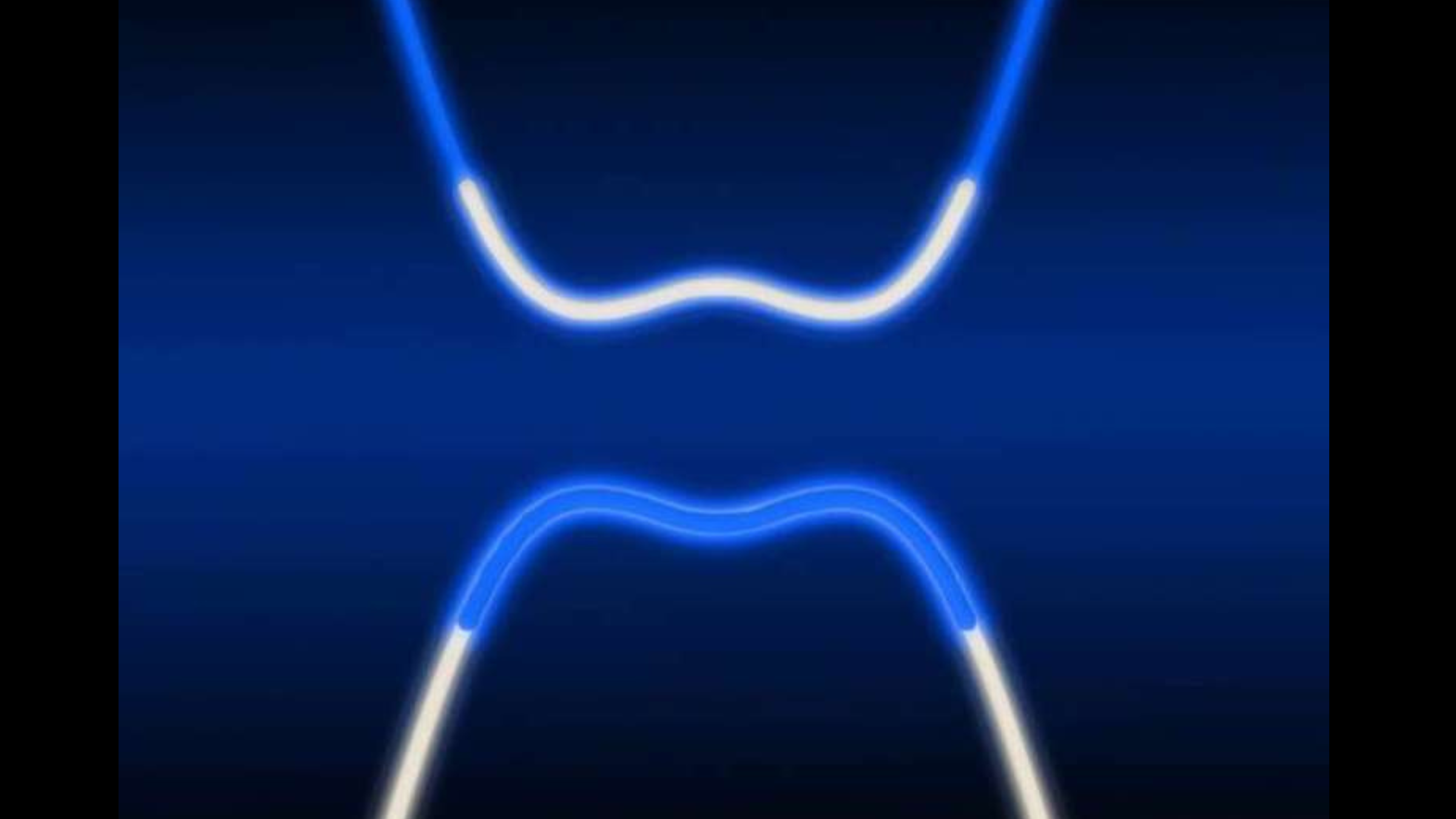
Courtesy: Scientists reveal the role of structural symmetry breaking in a candidate material for lossless energy transport, Baldini Lab
Scientists are searching for ways to detect and stabilize an exotic phase of matter called an excitonic insulator that could pave the way towards superfluid energy transport with no net charge (which is distinct from superconductivity). If realized, this phenomenon could lead to a new generation of devices where energy is transported at the nanoscale with minimal energy loss.
Earlier research suggested that the bulk crystal Ta2NiSe5 might be an excitonic insulator. Now, an international research team that includes Edoardo Baldini, assistant professor of physics at The University of Texas at Austin, has proven that it is not an excitonic insulator, and in the process settled a debate around what causes a particular phase transition in the material.
“Our experiments provide a new approach to identifying the driving force behind phase transitions in a wide range of candidate excitonic insulators,” said Baldini, lead author of a study published in the Proceedings of the National Academy of Sciences in April and former postdoctoral fellow at the Massachusetts Institute of Technology (MIT).
Spotting excitonic insulation in real solids has proven difficult so far. For the past two decades, it had been proposed that the quasi-two-dimensional solid Ta2NiSe5 may support an excitonic insulator phase above room temperature. Above a critical temperature (TC) of about 131° F (or 328 K), this material crystallizes in a layered structure that consists of parallel Ta and Ni chains. At TC, the system undergoes a semimetal-to-semiconductor transition, accompanied by a structural reorganization of the crystalline lattice. The scientific community has been engaged in an intense debate regarding whether this phase transition was induced by a purely electronic or a structural instability.
In the recently published study in PNAS, researchers in the U.S., Germany, and Japan probed the fundamental processes underpinning that transition via a joint experimental-theoretical approach. Using an advanced experimental tool called time- and angle-resolved photoemission spectroscopy under highly controlled conditions, they exposed Ta2NiSe5 to a tailored laser pulse and recorded a real-time movie of the fundamental components of the excitons (i.e., electrons and holes) as well as the structural degrees of freedom. To resolve these microscopic phenomena, the movie had to achieve an ultrafast time resolution of less than a millionth of a billionth of a second.
Tracking the dynamics of the material’s electronic and crystal structure after light excitation revealed spectroscopic fingerprints that are compatible only with a dominant order parameter of structural nature. In other words, the phase transition at higher temperatures is induced solely by structural instability. This implies that the changes in the crystal structure actually hinder the development of electronic superfluidity in this quantum material.
“This work demonstrates that Ta2NiSe5 is not an excitonic insulator and that dissipationless energy transport is hampered by the prominent rearrangement of the crystal structure,” said Nuh Gedik, professor of physics at MIT, who coordinated the research.
The findings were backed up by state-of-the-art calculations at several institutions, who combined different theoretical techniques to understand the microscopic origin of these changes in Ta2NiSe5 with unprecedented accuracy.
“Confirming the microscopic mechanism driving this transition to be structural in nature required highly demanding and intertwined electronic and structural modeling that also provided relevant information on the impact of possible excitonic contributions,” said Theory Director Angel Rubio from the Max Planck Institute for the Structure and Dynamics of Matter (MPSD) in Hamburg.
The groups of Eugene Demler at Harvard University, Andrew Millis at Columbia University, and Igor Mazin at George Mason University were partners in the theoretical collaboration. The experimental investigations were carried out at MIT, and the Ta2NiSe5 crystals used for this research were synthesized at the Max Planck Institute for Solid State Physics in Stuttgart and at the University of Tokyo.